Laboratory Tools in Science: Understanding Graduated Cylinders, Diagrams, and Repetition
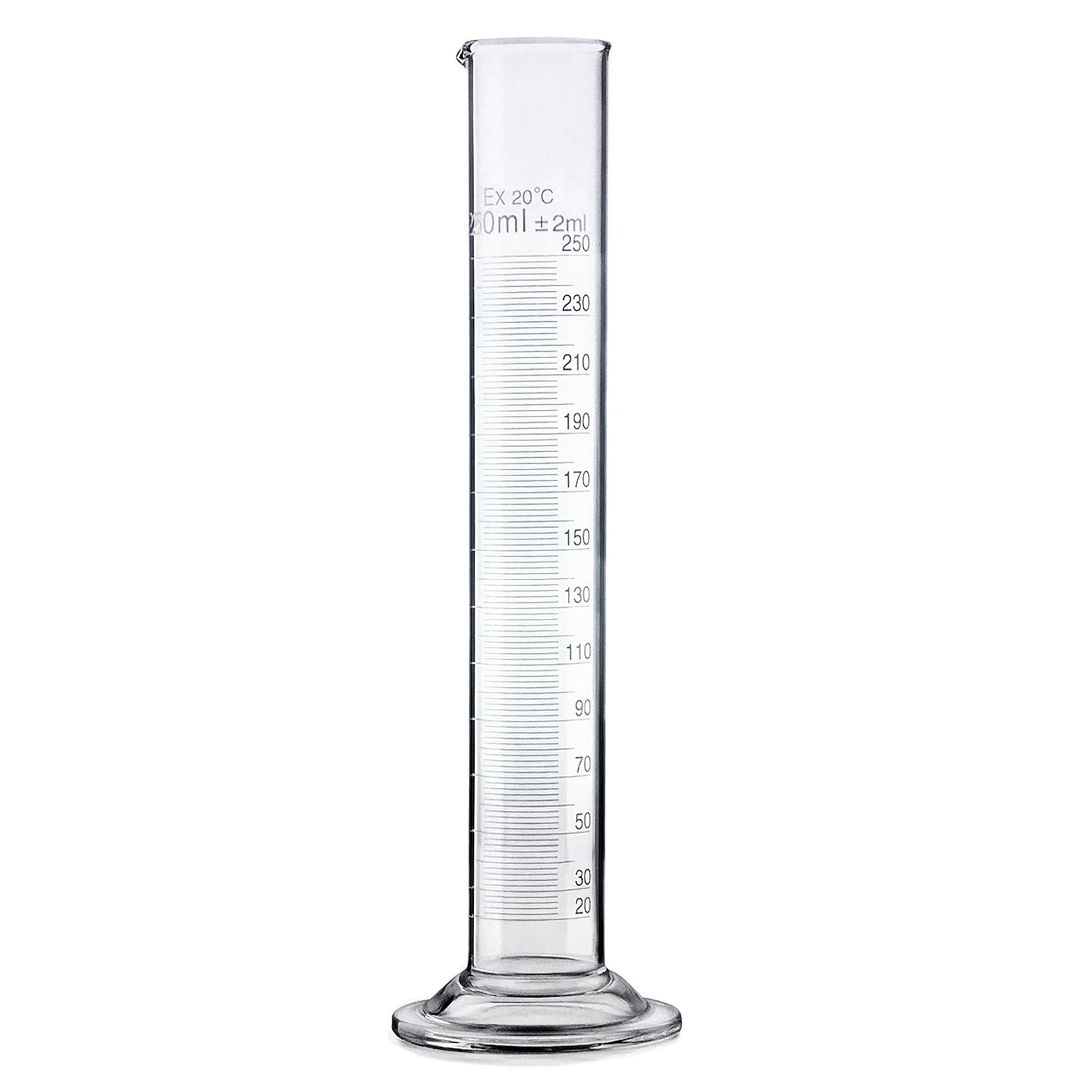
Laboratory tools in science: understand graduated cylinders, diagrams, and repetition
Science relies on precision, clear documentation, and verification to advance our understanding of the world. Three fundamental elements in scientific practice include graduate cylinders for accurate measurement, diagrams for visual representation, and repetition for result verification. Understand these tools and concepts is essential for anyone engage with scientific methodology.
Graduated cylinders: precision liquid measurement
A graduated cylinder stand as one of the virtually recognizable and oftentimes use tools in any laboratory setting. This tall, cylindrical vessel with marked volume increments serve a critical function in scientific experiments.
What’s a graduated cylinder?
A graduated cylinder is a narrow, cylindrical container make of glass or plastic with volume markings etch or print along its length. These calibrate markings allow scientists to measure liquid volumes with importantly greater accuracy than beakers or flasks.
The cylinder’s narrow design minimizes surface area relative to volume, reduce measurement errors cause by surface tension effects. Well-nigh graduated cylinders feature a stable base and ofttimes include a spout at the top to facilitate pour without spills.
Primary functions of graduated cylinders
Graduated cylinders serve several essential purposes in scientific work:
Precise volume measurement
The primary function of a graduated cylinder is to measure liquid volumes accurately. The narrow design and calibrated markings allow for measurements with precision typically range from 0.1 to 1 milliliter, depend on the cylinder’s size.
Scientists read the volume by observe the bottom of the meniscus (the curved surface of the liquid )at eye level. This technique ensure consistent and accurate readings across experiments.
Preparation of solutions
When prepare solutions of specific concentrations, accurate volume measurement become crucial. Graduated cylinders allow scientists to add precise amounts of solvents to dissolve substances, ensure the result solution have the intent concentration.
For example, when prepare a 0.1 molar solution of sodium chloride, a chemist must add precisely the calculated amount of water to the salt. Any deviation in volume would change the solution’s concentration and potentially affect experimental outcomes.
Liquid transfer
The design of graduate cylinders facilitates the transfer of measure volumes from one container to another with minimal loss. The pour spout help direct the flow of liquid exactly where need.
Density determination
By measure the mass of a know volume of liquid in a graduated cylinder, scientists can calculate density use the formula: density = mass / volume. This fundamental property helps identify substances and understand their behavior under different conditions.

Source: Cadbury.blob.core.windows.net
Types of graduated cylinders
Graduated cylinders come in various sizes and materials to suit different experimental needs:
Size variations
Common sizes range from 5 ml for measure small volumes to 2000 ml for larger quantities. The size selection depends on the volume range need for a particular experiment. Smaller cylinders typically offer greater precision for measure small volumes.
Material considerations
Glass graduate cylinders provide excellent chemical resistance and maintain their calibration over time. Yet, they break easy and pose safety risks in certain environments.
Plastic (oftentimes polypropylene )cylinders offer durability and resistance to breakage, make them suitable for educational settings and field work. Nonetheless, they may interact with certain solvents and can lose accuracy over time due to scratch or warp.
Specialized cylinders
Some graduated cylinders feature ground glass stoppers for storage of volatile liquids. Others have double scales for read different units or are design with special bases for increase stability during use.
Accuracy considerations
While graduate cylinders offer good precision, their accuracy have limitations:
Class a graduated cylinders meet higher calibration standards and provide greater accuracy than class b cylinders. For the virtually precise volume measurements, volumetric pipettes or burette surpass graduated cylinders in accuracy.
Temperature affect liquid volume through thermal expansion, so measurements should account for temperature variations. Many cylinders are calibrated at 20 ° c, and significant temperature deviations can introduce errors.
Diagrams in science: visual communication tools
Scientific diagrams serve as powerful visual tools that communicate complex information intelligibly and expeditiously. They bridge the gap between abstract concepts and tangible understanding.
What’s a scientific diagram?
A scientific diagram is a visual representation that illustrate structures, processes, relationships, or concepts in a simplify, organized manner. Unlike artistic illustrations, scientific diagrams prioritize clarity, accuracy, and informational value over aesthetic appeal.
Good scientific diagrams eliminate unnecessary details while emphasize key components relevant to the scientific concept being communicated. They oftentimes include labels, legends, scale indicators, and other elements that enhance understanding.
Types of scientific diagrams
Scientific disciplines employ various diagram types to communicate different kinds of information:
Structural diagrams
Anatomical diagrams show the structure and organization of organisms, from cellular components to organ systems. These diagrams help students and researchers understand spatial relationships between parts.
Molecular diagrams represent chemical structures, show how atoms bond unitedly to form molecules. These range from simple Lewis structures to complex three-dimensional representations of proteins or DNA.
Geological cross-sections illustrate the arrangement of rock layers and geological features beneath the earth’s surface, help geologists understand formation processes and resource locations.
Process diagrams
Flow charts depict sequential steps in a process, show how one stage lead to another. These are specially useful for illustrating metabolic pathways, experimental procedures, or decision trees.
Cycle diagrams illustrate recur processes like the water cycle, carbon cycle, or cell cycle. The circular arrangement emphasizes the continuous nature of these processes.
Mechanism diagrams show how components interact to produce a particular outcome, such as enzyme substrate interactions or the functioning of mechanical systems.
Relationship diagrams
Food webs illustrate energy transfer relationships between organisms in an ecosystem, show predator prey connections and trophic levels.
Phylogenetic trees display evolutionary relationships between species, help biologists understand common ancestry and divergence patterns.
Concept maps connect related ideas with label lines, show how different concepts relate to one another in a network of knowledge.
Data visualization
Graphs translate numerical data into visual formats, make patterns and trends more apparent. Common types include line graphs, bar charts, scatter plots, and pie charts.
Distribution maps show how variables are distributed across geographical space, such as species range, disease prevalence, or climate patterns.
Functions of scientific diagrams
Diagrams serve multiple crucial functions in scientific communication:
Simplification of complex information
Diagrams distill complex systems or processes into visually accessible representations. This simplification make challenging concepts more approachable without sacrifice essential information.
For example, the Krebs cycle in cellular respiration involve numerous chemical reactions, enzymes, and molecules. An intimately design diagram can present this complexity in a format that help students grasp the overall process while understand individual steps.
Spatial relationship clarification
Many scientific concepts involve spatial relationships that are difficult to describe use words solitary. Diagrams excel at show how components relate to each other in space.
Anatomical diagrams, for instance, show not upright what organs exist in the body but besides their relative positions, connections, and proportions — information that would require lengthy textual descriptions.
Process visualization
Dynamic processes become more comprehensible when visualize as a series of steps or stages. Diagrams can capture motion, transformation, or change over time in a static image.
The rock cycle in geology, for example, become lots clearer when depict as a diagram show how different rock types transform through various geological processes.
Communication across language barriers
Easily design diagrams can communicate scientific information across language barriers. While terminology may differ between languages, visual representations remain consistent, facilitate international scientific collaboration.
Create effective scientific diagrams
Effective scientific diagrams follow several key principles:
Clear labeling identify all significant components, with lines or arrow connect labels to the relevant parts without cross or create confusion.
Appropriate scale and proportion maintain accurate relationships between components, frequently include scale bars or indicators for reference.
Consistent use of symbols and colors help viewers interpret the diagram right. Legends explain what different visual elements represent.
Purposeful simplification remove extraneous details that don’t contribute to understand the target concept, focus attention on what matters.
Repetition in science: the foundation of reliability
Repetition stand as one of the cornerstones of the scientific method, ensure that discoveries represent genuine natural phenomena instead than artifacts, coincidences, or errors.
What’s repetition in science?
Scientific repetition involve conduct the same experiment or observation multiple times under control conditions to verify that results are consistent and reliable. This process take several forms, each serve a specific purpose in scientific investigation.
Repetition help distinguish between random variation and true effects, build confidence in findings, and allow for statistical analysis of results. It represents a fundamental safeguard against draw conclusions from anomalous or misleading data.
Types of scientific repetition
Scientists employ different approaches to repetition depend on their research goals:
Replication
Replication involve repeat an experiment use the same methods, materials, and conditions as the original. This direct repetition test whether the original results can be systematically reproduced.
Internal replication occur when the same researcher or research team repeat their own experiment to verify findings before publication.
External replication happen when different researchers at different institutions attempt to reproduce publish results, provide stronger validation of the findings.
Reproduction
Reproduction aim to achieve the same results use different methods or approaches. This test whether the findings reflect a genuine phenomenon quite than an artifact of a particular experimental setup.
For example, if a new drug show effectiveness in cell culture studies, researchers might reproduce the investigation use animal models and different measurement techniques to confirm the drug’s effects.
Multiple trials
Within a single experiment, scientists typically conduct multiple trials or measurements. This practice help account for random variation and experimental error.
By average results across multiple trials, researchers can obtain more reliable measurements and calculate statistical parameters like standard deviation to quantify variability.
The importance of repetition in scientific method
Repetition serve several critical functions in scientific research:
Reliability verification
Random events, measurement errors, or unusual circumstances can produce mislead results in a single experiment. Repetition help distinguish between genuine effects and statistical flukes.
If an effect persists across multiple repetitions, scientists gain confidence that they’reobservede a real phenomenon quite than an anomaly.
Error identification
Discrepancies between repetitions oftentimes signal problems in experimental design, execution, or interpretation. These inconsistencies prompt researchers to examine their methods more critically.
For example, if three trials yield similar results but a fourth differs dramatically, scientists might investigate whether equipment malfunction, whether procedures were followed aright, or whether some uncontrolled variable affect that particular trial.
Statistical power
More repetitions provide more data points, increase statistical power and allow for more robust analysis. This help researchers detect subtle effects that might not be apparent in limited samples.
Statistical techniques like ANOVA (analysis of variance )depend on multiple measurements to separate signal from noise in experimental data.
Establish boundaries and conditions
Repetition under varying conditions help establish the boundaries of scientific findings — determine when, where, and under what circumstances a phenomenon occur.
By consistently alter variables across repetitions, scientists can identify critical factors that influence outcomes and develop more comprehensive theories.
Challenges in scientific repetition
Despite its importance, repetition face several challenges in practice:
Publication bias
Academic journals typically prefer publish novel findings instead than replications of exist work. This creates a disincentive for scientists to conduct replication studies, potentially allow incorrect findings to persist unchallenged.
The” replication crisis ” n fields like psychology and biomedical research has highlight how publication bias can undermine scientific reliability.
Resource limitations
Repetition require additional time, funding, materials, and personnel. Resource constraints frequently limit how many repetitions researchers can perform.
Some experiments involve rare materials, expensive equipment, or unique circumstances that make repetition peculiarly challenging.
Complex systems
Some scientific investigations involve systems therefore complex or variable that exact repetition become virtually impossible. Examples include ecological field studies, certain astronomical observations, or studies of human behavior.

Source: helloartsy.com
In these cases, scientists must adapt their approach to repetition, focus on reproducible patterns instead than identical results.
Integration: how these elements work unitedly
Graduated cylinders, diagrams, and repetition don’t exist in isolation but work unitedly synergistically in scientific practice.
Precise measurements from tools like graduate cylinders generate reliable data that can be visualized through diagrams and validate through repetition. The diagram of an experimental setup might include graduate cylinders, while repetition confirm that measurements take with these cylinders are consistent.
Unitedly, these elements form part of the infrastructure that support the scientific method’s goal of produce reliable, verifiable knowledge about the natural world.
Conclusion
Graduated cylinders, scientific diagrams, and repetition represent fundamental tools and practices in scientific methodology. The graduated cylinder exemplifies how specialized equipment enable precise measurement, while diagrams demonstrate the power of visual representation in communicate complex information. Repetition underscore the self correct nature of science, where verification and validation remain essential to build reliable knowledge.
Understand these basic elements provide insight into how science operate as a discipline, systematic approach to discovery. From elementary school classrooms to advanced research laboratories, these principles guide scientific inquiry and help transform curiosity into knowledge.
As science continue to advance, the fundamental importance of accurate measurement, clear visual communication, and rigorous verification remain constant — a testament to the endure value of these basic scientific tools and practices.